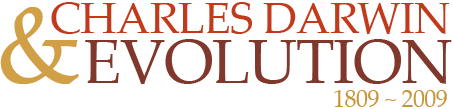
Darwin's theories depended on there being a way for offspring to inherit their parents' characteristics. For example, if a particular giraffe has a longer neck than average, allowing it to reach higher branches of trees and access extra food, it may be more successful than other giraffes. Unless this longer neck is passed on to its children, though, it would be an evolutionary dead end - natural selection operates by the most successful individuals passing on the traits which make them successful to the next generation. It is obvious that traits can be inherited, since children usually resemble their parents. For thousands of years, humans have bred only the most productive and amenable individuals among domesticated animals and plants so as to encourage these beneficial traits in their offspring.
The Discovery Of DNA
Mendel's experiments showed the principles behind inheritance of traits, but the actual process of inheritance remained a mystery. Several people, including the German botanist Wilhelm Hofmeister, had previously observed distinct thread-like shapes within dividing cells, termed chromosomes (meaning "coloured bodies", since they were coloured when stained with dyes). These separate from each other as the cell divides, resulting in one full set of chromosomes in each resulting cell. The chromosomes were suspected to be involved in inheritance, but no-one knew exactly how that might be.
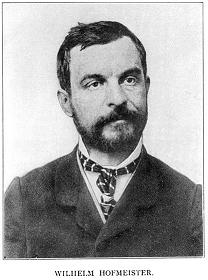
Chromosomes are formed of long strands of DNA wrapped around proteins called histones. DNA had been found in 1869 by the Swiss biochemist Friedrich Miescher, who termed it "nuclein", and was further studied in the early 1900s. It consists of four different units, termed bases, which are linked together in long chains. As there are only four different bases (adenine, thymine, cytosine and guanosine), it seemed hard to imagine how DNA could store the complex information needed for inheritance. However, Hershey and Chase showed in 1952 that DNA was the hereditary material for bacteriophages, viruses which infect bacteria.
The Double Helix
The discovery of the structure of DNA was crucial for understanding how it was involved in inheritance. X-ray crystallography allows the structures of molecules to be studied based on the way they scatter X-rays, and was crucial to this development. Rosalind Franklin had taken X-ray images of DNA molecules which were seen by two Cambridge scientists, James Watson and Francis Crick, allowing them to realise that DNA consisted of two long strands wound round each other to form a double helix.
They worked on models of the four bases of DNA, trying to fit them together in a logical way to form the double helix. The structure they proposed in 1953 involved each of the bases on one strand pairing with a particular base on the other strand, so that adenine always paired with thymine and cytosine was always opposite guanosine. This model was accurate, and it was soon discovered that each set of three bases along the chain coded for one amino acid, the building blocks of proteins.
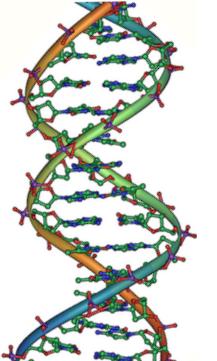
DNA & Evolution
This genetic code solved the problem of how four bases could be sufficient to encode hereditary information, and the structure of DNA explained how cells pass on their genetic information. Since bases always pair with their partner on the other strand of the DNA double helix, one of the two strands is sufficient to act as a template for a new strand. An identical copy of each DNA molecule can be created, then segregated to each cell. In the case of sexual reproduction, one set of chromosomes from each parent is passed on to the offspring, each acting as a partial 'blueprint' for the new organism. Any minor errors in copying may lead to mutations, which could give organisms a selective advantage or disadvantage.
An understanding of how DNA allows inheritance has meant that Darwin's theory of natural selection can now be understood on a molecular level, and evolution can be studied by analysing changes to organisms' DNA. Amongst many other applications, similarities and differences in shared DNA sequences between related species allow their evolutionary relationship to be explored and represented in a phylogenetic "tree"; it is often found that even distantly-related organisms share common evolutionary ancestors.
From Gene To Genome
The discovery of the structure of DNA and the figuring out of the amino acid code meant if biologists could sequence a stretch of DNA they could read it to figure out what it did. Within two decades of Watson and Crick’s discovery, methods had been established to sequence long stretches of DNA. Early results were quite surprising; for example one team sequenced a long stretch of the same region of a chromosome from humans and chimps and to everyone’s surprise the two were almost identical. Quickly people realised the potential of sequencing genomes, and by the 1980s the idea was floating about to sequence the human genome. Sequencing methods were still laborious and slow, but with new methods and an international collaboration this feat was achieved in 2004.
There are now major projects to sequence genomes of many different living species to cover the tree of life. New methods are continuously being developed and genomes are sequenced more and more rapidly. By 2007 the genomes of 1879 viruses, 577 bacterial and 23 eukaryotes (organisms made of cells with nuclei) had been sequenced, the numbers now almost doubling each year. Genomics provides some of the best evidence for evolution through common ancestry; we can find the some of the same genes in bacteria that we find in humans for example. We can also look at what functions these genes perform, and how these functions have evolved. One example is that of the Hox genes which you can read about in the case studies section.
Written by Chris Hurley
References & Further Reading
An Intelligent Person's Guide to Genetics
by Adriaan Woolfson, Duckworth Overlook: 2004
The Double Helix
by James D. Watson, Weidenfeld & Nicolson: 1968
Evolution: The History of an Idea
by Peter Bowler, University of California Press: 1983
The Evolution of Darwinism
by Timothy Shanahan, CUP: 2004
This Is Biology
by Ernst Mayr, Harvard University Press: 1997
The Language of the Genes
by Steve Jones, Happer Collins: 1993